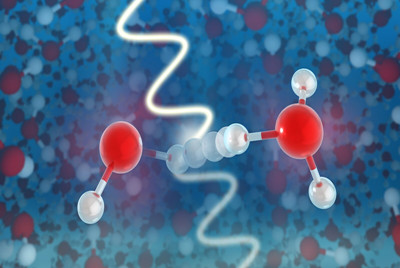
An international team led by Argonne has visualized the elusive, ultrafast proton transfer process following the ionization of water.
Understanding how ionizing radiation interacts with water—like in water-cooled nuclear reactors and other water-containing systems—requires glimpsing some of the fastest chemical reactions ever observed.
In a new study from a worldwide collaboration led by scientists at the U.S. Department of Energy's (DOE) Argonne National Laboratory, Nanyang Technological University, Singapore (NTUSingapore), the German research centerDESY, and conducted atSLACNational Accelerator Laboratory, researchers have witnessed for the first time the ultrafast proton transfer reaction following ionization of liquid water.
The proton transfer reaction is a process of great significance to a wide range of fields, including nuclear engineering, space travel and environmental remediation.The observation was made possible by the availability of ultrafast X-ray free-electron-laser pulses, and is basically unobservable by other ultrafast methods. While studying the fastest chemical reactions is interesting in its own right, this observation for water also has important practical implications.
"The truly exciting thing is that we've witnessed the fastest chemical reaction in ionized water, which leads to the birth of the hydroxyl radical," said Argonne distinguished fellow Linda Young, the senior corresponding author of the study."The hydroxyl radical is itself of considerable importance, as it can diffuse through an organism, including our bodies, and damage virtually any macromolecule includingDNA,RNA, and proteins."
By understanding the time scale for the formation of the chemically aggressive hydroxyl radical and, thereby, gaining a deeper mechanistic understanding of the radiolysis of water, it may ultimately become possible to develop strategies to suppress this key step which can lead to radiation damage.
When radiation with sufficient energy hits a water molecule, it triggers a set of virtually instantaneous reactions. First, the radiation ejects an electron, leaving a positively charged water molecule (H2O+) in its wake. H2O+is extremely short-lived—so short-lived, in fact, that it is virtually impossible to see directly in experiments. Within a fraction of a trillionth of a second, H2O+gives up a proton to another water molecule, creating hydronium (H3O+) and a hydroxyl (OH) radical.
Scientists had long known of this reaction, with a first sighting in the1960s when scientists at Argonne first detected the electron ejected from water by radiolysis. However, without a sufficiently fast X-ray probe like that provided by the Linac Coherent Light Source (LCLS) atSLAC, aDOEOffice of Science User Facility, researchers had no way to observe the residual positively charged ion, the other half of the reaction pair.
"Being part of this highly collaborative and world-class group was just as exciting as watching water molecules dance in slow motion following ionization," saidSLACinstrument scientist Bill Schlotter, who with Young led the conceptual design of the experiment."The keys to capturing the water in action are the ultrashort X-ray pulses atLCLS. By adjusting the'color' of these X-ray pulses, we can distinguish between the specific ions and molecules that participate."
The"freeze-frame" technology offered byLCLSoffered researchers the first opportunity to watch the time evolution of the hydroxyl radical. While according to Young, the researchers would have liked to isolate the spectroscopic signature of the H2O+radical cation as well, its lifetime is so short that its presence was only inferred from theOHspectroscopy measurements.
The ultrafast proton transfer that creates the hydroxyl radical gives rise to a special spectroscopic signature that indicates the rise of the hydroxyl radical and is a"time stamp" for the initial creation of the H2O+. According to Young, the spectra of both species is accessible because they exist in a"water window" where liquid water does not absorb light.
"The major accomplishment here is the development of a method to watch elementary proton transfer reactions in water and to have a clean probe for the hydroxyl radical," Young said."No one knew the time scale of proton transfer, so now we've measured it. No one had a way to follow the hydroxyl radical in complex systems on ultrafast timescales, and now we have a way to do that as well."
Understanding the formation of the hydroxyl radical could be of particular interest in aqueous environments containing salts or other minerals that might, in turn, react with ionized water or its byproducts. Such environments could include nuclear waste repositories or other places in need of environmental remediation.
NTU experiments are conducted using tabletop laser equipment for fundamental chemistry, which led to the collaboration with Argonne and DESY. Credit: NTU Singapore
The development of the theory behind the experiment was led by Robin Santra of the Center for Free-Electron Laser Science atDESYin Germany. Santra showed that through ultrafast X-ray absorption, scientists could detect the structural dynamics—both in terms of electron and nuclear motion—near the ionization and proton transfer site.
"We could show that the X-ray data actually contain information on the dynamics of the water molecules that enable the proton transfer," said Santra, who is a lead scientist atDESYand principal investigator at the Hamburg Centre for Ultrafast Imaging, a cluster of excellence at the University of Hamburg andDESY."In just50quadrillionths of a second, the surrounding water molecules literally move in on the ionized H2O+until one of them comes close enough to grab one of its protons in a sort of handshake, turning into hydronium H3O+and leaving behind the hydroxyl radicalOH."
This work was motivated by earlier research by Zhi-Heng Loh fromNTUSingapore, the lead author and co-corresponding author for this paper.
"Since joiningNTUnine years ago, I and the members of my group have been studying the ultrafast dynamics that accompany the ionization of molecules, both in the gas phase and in aqueous medium, using femtosecond laser pulses spanning the infrared to the extreme ultraviolet. Our earlier work on ionized liquid water provided a glimpse of the lifetime of the H2O+radical cation, albeit via indirect probing in the near-infrared," Loh said."We realized that a definitive experiment to observe the H2O+radical cation would require soft X-ray probing, which however, lies beyond the capability of most tabletop femtosecond light sources. So when Linda approached me after hearing my talk on ionized water at a meeting in2016, and wanted to collaborate on an experiment at theLCLSX-ray free-electron laser, I was absolutely thrilled."
A paper based on the study,"Observation of the fastest chemical processes in the radiolysis of water," will appear in the January10online issue of Science.